The Future of Space, Part I: The Setup
This is the first part of a two-part series on the future of the space industry. Although I’ve followed the space for a while now, I learned a lot in the process of writing this series. Part 2 is the real fun one on the near-term future of space. It should be finished in a few weeks.
Expansion of life across our solar system and beyond has been a dream of mine since childhood. Of course, this isn't uncommon among other sci-fi enthusiasts, or anyone who grew up knowing we've sent humans to the Moon but haven't sent them back in nearly 50 years.
Space is fascinating for many reasons. It's a frontier in every sense: physically, technically, even socially. It's at the bleeding edge of what humanity is capable of. "Looking to the stars" and "shooting for the moon" are common idioms because space has defined our limits for generations.
Now (finally!) the technical and business tailwinds are coming together to make it possible. The cost and ease of getting to space are about to improve by many orders of magnitude. This will drive the space industry to be one of the biggest sources of growth over the next 10-20 years.[1] It will make existing technologies cheaper and more ubiquitous, like allowing worldwide high-speed internet in even the most remote, rural areas. It will also open up a host of new possibilities previously only imagined in science fiction.
This is the first of a two-part essay on the upcoming future of the space industry. I've been closely following SpaceX's progress in particular since their first launch of the Falcon 9 in 2010, so I'm excited to finally write about it.
Why now?
TLDR: SpaceX has pushed cost to orbit down by 10x, and will by another 10x in 5 years. Along with further commercialization and government funding, a threshold has been crossed.
The success of commercial launch services puts the space industry in the same place as the early days of railroads in the 1800s or commercial ocean shippers in the 1600s. The key here is early days as things are really just getting started.
The "why now?" can be reduced to one chart — the average cost to get 1 kilogram to orbit:
In the next section I'll go over the reasons why this makes such a big difference. But first, how did it happen? As should be evident by the chart, this is essentially the story of one company — SpaceX.
The driving ambition for Elon Musk when he founded SpaceX in 2002 was to drastically reduce the cost of escaping Earth's gravity. Their "MVP" was the Falcon 1, a single-engine rocket that could launch small satellites. Falcon 1 only launched 5 times, with only the last 3 succeeding. Haven proven viability, SpaceX quickly moved onto production of the Falcon 9, a scaled up version with nine Merlin engines eventually capable of delivering over 22,000 kg to Low Earth Orbit (LEO). Here's the price progression of each SpaceX rocket, starting from the base of what a conventional rocket costs:
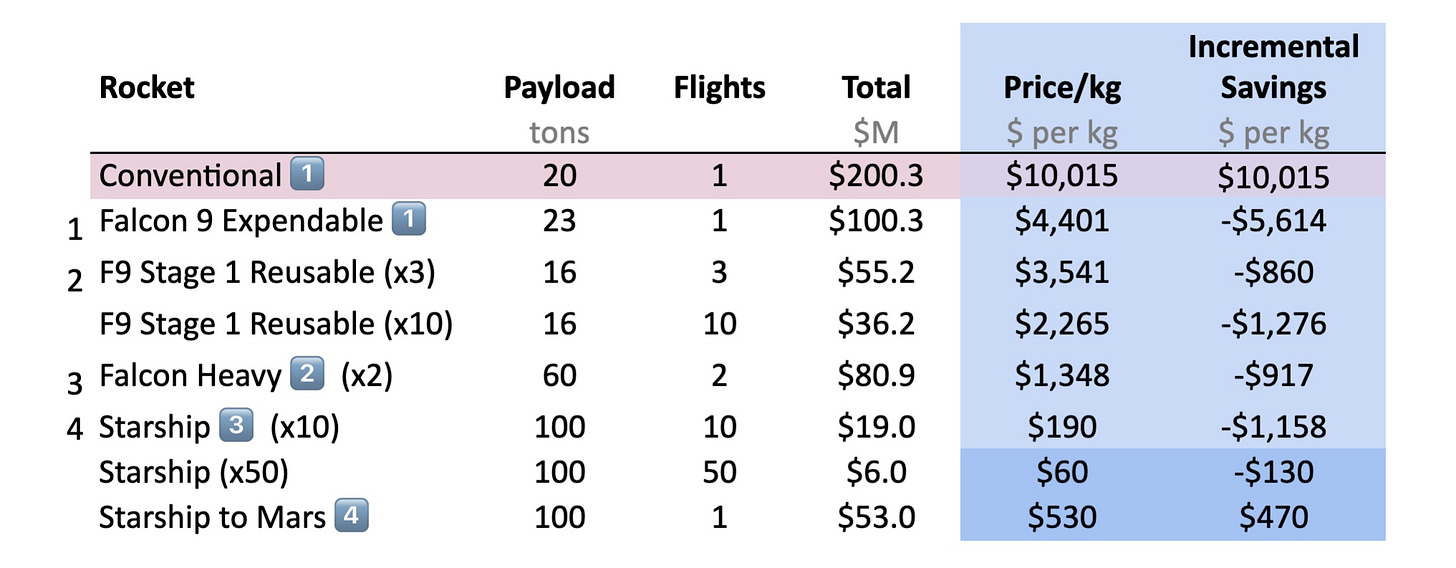
Driving the first order-of-magnitude reduction in cost are the following:
Engineering from first principles. Designed and engineered from the ground up, famously using first principles to rethink standard industry practices. This led to seemingly trivial savings like using ethernet cables rather than serial cable bundles. But added up they make a huge difference.
Better incentives. Traditional government contracts were cost-plus. This incentivizes contractors to increase their costs both to make more profit and for more admin overhead to track expenses. With fixed-prices, companies are incentivized to drive costs down as much as possible.
Standardization of launch config. Rather than customized configurations for each launch and customer, SpaceX "productized" the Falcon 9, allowing for cheaper setups and repeated processes.
Reusability. Why is air travel cheaper than space travel? It's obvious, right? Aircraft are reusable while rockets are destroyed after a single use. But not anymore, as anyone not living under a rock now knows that SpaceX can land and reuse the first stage(s) of their rockets.
And the next 10x reduction with Starship:
Bigger rocket. There are economies of size in rocketry: The bigger the rocket, the higher the payload-to-fuel ratio can be.
Full flow combustion cycle engine. This higher-complexity engine design makes it more efficient and capable of being reused many times with very little maintenance.
Lower-cost methane as fuel. Methane is cheaper than the previously used RP1 (rocket fuel), and SpaceX is planning on literally drilling for methane gas on their Texas property and synthesizing it on their converted oil rigs. (It can also be synthesized on Mars...)
Full reusability. 100% of Starship will be reusable, allowing dozens (or hundreds?) of uses for each stage and engine.
More launches. The more launches you can sell in a year, the less markup you need to charge to cover admin costs. Economies of scale and purchasing power are also achieved in raw materials and fuel production.
Refuel in orbit. Starship can park in orbit while it's refueled by up to 8 other launches. This makes payload capacity to orbit the same as payload capacity to nearly anywhere in the solar system. Imagine what we can do with the ability to send over 100 tons to the Moon, Mars or Europa.
Government funding, particularly from NASA, has been a key enabler. Without these contracts it would have been very difficult for SpaceX to fund R&D. And they'll continue to play a key role for SpaceX and other commercial space providers. In recent years NASA has stepped up their commercial contracts significantly, and with further falling costs this is likely to continue. (See footnote [2] for a list of recent milestones.)
This moment for space companies is the equivalent of 1995 when the NSF dropped all restrictions on Internet commerce, which let private companies take over the backbone. The breaking of the dam that releases a tidal wave of activity.
The cost-driven industry flywheel
Expensive launches aren't just costly in their own right — they lead to cost inflation of everything else. If it costs $100M to get a satellite to orbit, reducing the cost of development from $10M to $5M is only a 5% difference. So why not over-engineer, paying up for components and testing to ensure everything is perfect? Now if a launch costs $10M, there's more incentive to cut costs. Even if there's an issue, a second launch is much cheaper. Order-of-magnitude-lower launch costs will lead to similar decreases in payload costs.
From a Morgan Stanley report:
Currently, the cost to launch a satellite has declined to about $60 million, from $200 million, via reusable rockets, with a potential drop to as low as $5 million. And satellite mass production could decrease that cost from $500 million per satellite to $500,000.
More launches will lead to even cheaper costs, which will lead to cheaper payloads, which... see where I'm going here?
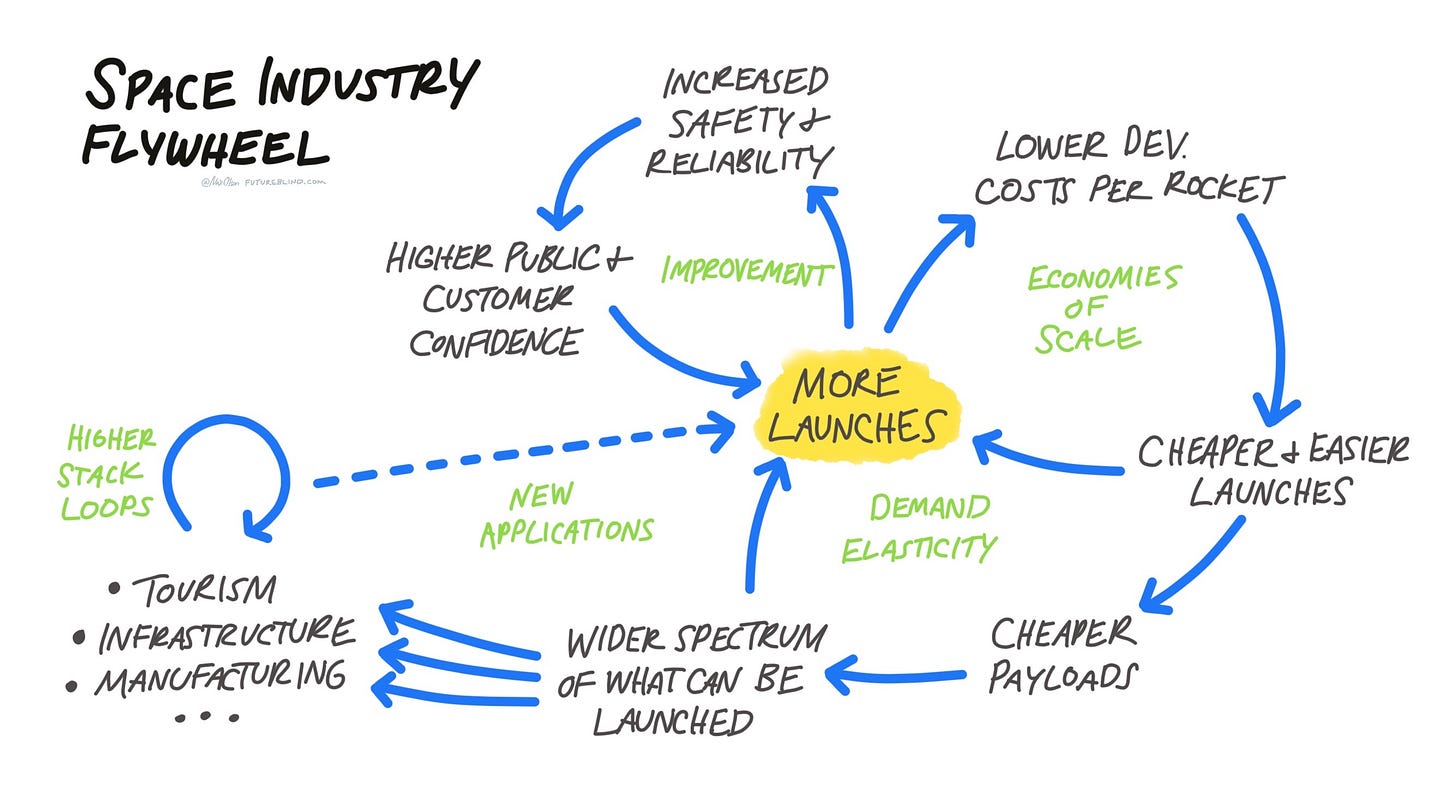
SpaceX has initially started the flywheel that got the industry to this inflection point.[3] But it won't be the only one turning it. Ultimately to truly take advantage of space transportation we'll be seeing many competing service providers, at all different levels of payload size and capability.
The flywheel is already turning and has led to a higher volume of launches:
At some point in the near future we’ll be seeing a launch per day, with spaceports treated more like shipping ports: hubs of travel and commercial activity.
Current state of the industry
Before moving on to Part II, I want to quickly review the two main categories of payload currently being launched:
Government research and exploration.
International Space Station cargo. In the U.S. this encompasses missions for Commercial Resupply (sending equipment and supplies) and Commercial Crew (sending people).
Other research and exploratory efforts. This includes missions like the recently landed Mars Perseverance rover, the James Webb Telescope set to launch after much delay later this year on an Ariane 5 rocket, and the Europa Clipper set to launch in 2024.
Satellites. Communication and Imaging satellites account for a vast majority of the space industry. Exploratory missions get all the publicity, but they are currently very tiny. This will continue, especially with broadband internet constellations.
The use of communication satellites in particular is already a ubiquitous part of everyday life: from GPS navigation[4] to phone calls, TV signals, internet, and more. Satellite imagery as well: what once was a tool for only the military and intelligence agencies of large governments is now used by anyone with a smartphone.
Satellites come in a range of sizes, from tiny CubeSats the size of a shoebox launched 100s at a time; to huge geostationary satellites that take up the entire payload of a rocket.[5] Most of this hardware — particularly for the larger ones — requires costly, sophisticated engineering and infrastructure. The full stack can include satellite manufacturers, operators, suppliers, and ground equipment. As costs come down, so will satellite size and launch frequency.
What's to come
I hope I've convinced you that getting to space is about to get a whole lot easier.
In Part II, I'll talk about the progress we will potentially see in space in the upcoming 10 to 20 years: commercial space stations, tourism, manufacturing, mining, exploration and more.
Footnotes
The same is true for biotech in the upcoming decades. Areas like AI and Crypto will play big roles as well, but they're not the thing. They're the "thing that gets us to the thing".
Here's a timeline of a few milestones:
2008-12 — Commercial Resupply Services (CRS) contract of $1.6B to SpaceX and $1.9B to Orbital Sciences to deliver supplies to ISS. This helps fund Falcon 9 development.
2012-05 — SpaceX Dragon capsule launches "empty" to perform tests and dock with the ISS, the first commercial spacecraft ever to do so.
2012-10 — SpaceX CRS-1 mission sends Dragon with supplies to ISS. Dragon is the only cargo vehicle at the time capable of returning supplies to Earth.
2014-09 — NASA awards final Commercial Crew Program (CCP) contract to SpaceX ($2.6B) and Boeing ($4.2B) for the capability to send 4-5 astronauts to the ISS. First flights for both initially planned in 2017.
2020-01 — NASA awards Axiom Space the first ever contract to build a commercial module for the ISS.
2020-04 — NASA awards lunar lander contracts to Blue Origin, Dynetics, and SpaceX under the Artemis program. The goal is to land "the first woman and the next man" on the Moon by 2024.
2020-05 — Commercial Crew Demo mission sends 2 astronauts to ISS. These are the first astronauts on a commercial mission, and the first from US soil since retirement of the Space Shuttle in 2011. 10 million people worldwide watched it live.
2020-11 — Crew 1, the first operational flight, sends 4 astronauts to ISS. Due to delays and other issues, Boeing's Starliner isn't set to fly for another year.
2020-12 — NASA awards Blue Origin a Launch Services contract to transport planetary, Earth observation, exploration and scientific satellites.
Elon Musk is a master at many things, but one of the greatest is his ability to get massive, company- or industry-wide flywheels moving.
Global Positioning System (GPS) was developed by the military in the 1960s but not made public until 1996. GPS is an extremely critical part of our current technical infrastructure. Every time you use your phone to navigate, order food, or track your run, it is pinging multiple GPS satellites to triangulate your exact location.
Here's a good visual size comparison of satellites: